Speculating on Tritium Futures
Why Defense Material Should Fuel Fusion Innovation
Brief
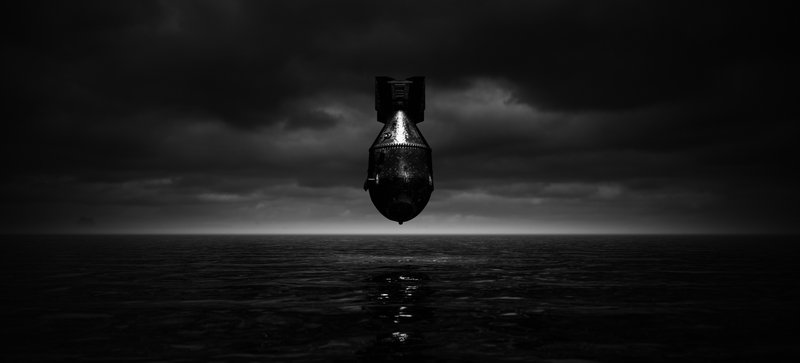
Shutterstock
April 24, 2023
This brief is part of a series by New America’s Nuclear Futures Working Group, which brings together emerging researchers from academic, government, advocacy, and policy spaces to develop research on nuclear security policy problems through the lens of a changing global environment.
Executive Summary
Nuclear fusion is commonly achieved by fusing together two isotopes of hydrogen, tritium and deuterium, under high pressures and temperatures. In nuclear weapons, this process “boosts” the explosive yield of uranium and plutonium by 10-100x, and in experimental reactors, it produces substantial amounts of heat that can be used to generate electricity. Most of the world’s tritium reserves and production capacity are dedicated for use in nuclear weapons, and what remains for peaceful applications is precarious. Since tritium cannot be stockpiled due to its relatively short half-life of 12.3 years, it must be continuously produced. Presently, defense and commercial tritium supply chains are kept segregated, and only the former is readily scalable. Tritium is both an irreplaceable component in virtually all modern weapon designs and an essential fuel material for nascent nuclear fusion technologies that may one day provide sustainable, low-carbon energy solutions.
A holistic national tritium strategy leveraging defense production capabilities to support the uncertain and dwindling fusion fuel supplies with reliable defense production would provide many advantages. The first nations to commercially deploy fusion would be the global leaders and play a significant role in establishing norms. Additional low-carbon energy options would support long-term climate change mitigation. By reducing reliance on dual-use technologies such as centrifuges for uranium enrichment, the transition from fission to fusion energy would also reduce nuclear proliferation risk. Cultivating domestic supply chains for energy production would reduce dependence on autocratic regimes and further strengthen national security. Finally, when the world reverses current proliferation trends and renews disarmament efforts, valuable tritium reserves can be more readily put to productive and peaceful use. The United States and the world should not wait for future geopolitical stability to implement practical strategies at this critical technology nexus.
Policy Recommendation: Gigatons-to-Gigawatts
- Develop and implement a national strategy facilitating the military-to-civilian transfer of tritium for the development and sustainment of nuclear fusion technology.
- Support first-of-a-kind fusion reactors during experimental and demonstration phases of development and bridge the gap until such reactors become self-sustaining through in situ tritium breeding.
- Attract more actors and capital investments in the fusion research and development pipeline by increased tritium availability.
- Establish a unilateral framework focused on sharing domestic military production and reserves and serve as a model for future bilateral or multilateral agreements.
Background
Fusion Technology in the Real World
With the recent breakthroughs in fusion technology, researchers and science journalists are confronting the material requirements of a vaporware promise turned hardware reality. A significant limiting factor for the viability of fusion energy is the continued availability of a rare isotope of hydrogen known as tritium, which is used to fuel fusion reactors. Amit Katwala with Wired and Daniel Clery with Science have written about a “fuel crisis” and fear that fusion may be “out of gas” before it even begins. Not all designs currently under development require tritium, but the fusion of deuterium and tritium (DT fusion) remains the most appealing in principle. Since deuterium, another isotope of hydrogen, is relatively cheap and plentiful, tritium is the primary fuel concern. For the foreseeable future, fusion technologies based on DT fusion will likely be the most feasible, that is, if that future can sufficiently increase tritium production capacity to sustain development and deployment.
This is not nuclear power’s first “fuel crisis.” In the 1960s and 70s, during the early decades of fission power, many experts estimated that uranium was a rapidly depleting resource. They worried that nuclear power would have no future unless it transitioned to breeder reactors and a closed plutonium fuel cycle. However, this transition never occurred. Conveniently, the uranium abundance in Earth’s crust was greatly underestimated, and there continues to be no resource pressure to switch to plutonium for nuclear fuel.
The Ins and Outs of Tritium
As a fuel material, tritium is markedly different from uranium in almost every aspect. Instead of decaying over billions of years, tritium has a radioactive half-life of just 12.3 years. It exists at a sufficiently low natural equilibrium in the global environment that there is no economic way to extract and purify it.
Virtually all of the world’s usable tritium is produced either incidentally in heavy-water reactors as a byproduct or intentionally in light-water reactors using specially designed targets. The former method is dominated by detritiating coolant from an aging fleet of CANDU heavy-water reactors with the bulk controlled by Canada and dedicated for peaceful use in the ITER fusion project in France. The latter method has been predominantly used in military production reactors, which also produce weapons-usable plutonium. In a notable exception to this trend, the United States has been producing tritium for the past two decades in commercial light-water reactors at the Watts Bar facility operated by the Tennessee Valley Authority (TVA), a federally owned utility.
The vast majority of all tritium reserves are currently used in and dedicated to thermonuclear weapons. As little as 2 grams of tritium can boost the fission yield of a weapon by an order of magnitude. In practice, this means that the masses of plutonium and uranium required to achieve the desired explosive power can be significantly lowered, resulting in much smaller and lighter warheads. Additionally, the ability to modulate the DT fusion process in situ allows for an entirely new class of selectable-yield weapons (so-called “dial-a-yield”). These properties ensure the central role of tritium in all but the most rudimentary nuclear weapon programs.
Due to tritium’s short half-life, resulting in ~5 percent/year lost to decay, each warhead requires on-going life cycle maintenance to ensure that sufficient quantities remain to meet design specifications. Without on-going production to replenish tritium, any thermonuclear weapon stockpile would be neutralized or substantially diminished over time as a direct function of decay. In the words of one National Nuclear Security Administration (NNSA) Field Office Manager, “without tritium, there wouldn’t be a nuclear stockpile.”
Ambivalent Atoms
A common element of Katwala’s and Clery’s pessimistic prognostications on tritium availability is an acknowledgement that nuclear weapon states (NWS) possess significant stockpiles and production capacities for military use, but sharing these reserves is summarily ignored or dismissed as “unlikely.” Neither author clearly explains why defense material should be considered off limits, but the assumption seems to be that tritium requirements for nuclear weapons are carefully calibrated and could not be infringed upon without detracting from national security. Whether or not this is true, the “swords to plowshares” sentiment of Dwight D. Eisenhower’s landmark “Atoms for Peace” address to the United Nations—in which he proposed a vision of peaceful applications and distribution of nuclear energy around the world—appears to have lost some resonance since its delivery in 1953. Furthermore, the seemingly reflexive dismissal of the prospect of sharing reserves belies two salient historical precedents: (1) the market for tritium was kickstarted in 1959 when Oak Ridge National Labs (ORNL) made 100g of tritium in excess of defense requirements available for purchase and commercial use and (2) the most successful reduction in weapons-grade highly-enriched uranium (HEU) stockpiles, the Megatons-to-Megawatts program, was itself a military-to-civilian transfer of weapons material for energy production, albeit one focused on disarmament.
Before ORNL opened the floodgates, the entire global tritium market was only several grams and mostly limited to research applications. Radioluminescence, a reliable light-source requiring no external power, is a key commercial application for driving tritium demand. Tritium is also a much safer alternative to radium, which is notorious for the poisoning and deaths of many “Radium Girls” who had hand-painted glow-in-the-dark watch dials. Currently used in remote airport runway lighting, exit signs, and even gunsights, the commercial market for tritium has steadily grown. Tritium also plays an important role in nuclear medicine as a tracer material. More recent numbers show that ORNL is making available ~200g/year for trade, and Mb-Microtec, the parent company of Trigalight and one of the highest users of commercial tritium, processes ~40g/year.
The Megatons-to-Megawatts program was a bilateral agreement between the United States and Russia where ~500 metric tons of Russian HEU was down-blended into fuel suitable for U.S. light-water reactors. This blended low-enriched uranium (BLEU) fuel provided up to 10 percent of total U.S. electricity demand. This effort successfully eliminated significant stockpiles of HEU, helped generate vast amounts of low-carbon energy to mitigate climate change, and sequestered the remaining spent fuel in the U.S. open fuel cycle. Irina Wang’s provocative retrospective on this program calls for a new “weapons-to-energy policy” to once again harmonize our disarmament and climate agendas.
It is time to imagine a new program and further advance the promise of “Atoms for Peace.” Given fusion’s significant increase in energy density over fission, such a program might be called "Gigatons-to-Gigawatts."
Gigatons-to-Gigawatts
The case for creating a Gigatons-to-Gigawatts program is threefold.
First, it will directly address the impending fuel crisis facing fusion technology. Any nuclear fusion technology that uses tritium fuel will need to ultimately breed much of its own tritium to be sustainable. Clery aptly lays out in his article near-term tritium supply challenges for fusion research and development on the path to sustainability. He projects that the Canadian tritium stockpile could narrowly sustain an estimated requirement of 1-2kg/year for fusion research over the next two to three decades. However, some tritium capacity currently dedicated to military use could mitigate supply uncertainties and bolster confidence in capital investments during the research and development phase. A much bigger problem emerges once a 3-gigawatt fusion reactor needs to rely on unproven tritium breeding technologies to replace an estimated 167kg/year. The best estimates suggest that breeding might produce 105 to 115 percent of the initial fuel used. Being able to hedge the supply with military tritium would backstop any commercial breeding shortfalls, even if it fell below 100 percent. This would allow for the necessary technologies to be refined during the early years of operation without dooming the entire enterprise due to modest inefficiencies.
Tritium production capabilities in fission reactors can support, though not fully supply, fusion operation, but there are limitations. While the United States has demonstrated that tritium production can be incorporated into the current fleet of civilian reactor designs without significant core alterations, it is not achievable without some trade-offs. Most notably, tritium production requires increased fuel use to achieve the same power outputs with the same fuel cycle durations. This results in not only an increased volume of spent fuel but also measurably higher tritium releases to the environment. In the case of Watts Bar, concerns regarding such releases necessitated the construction of a 500,000-gallon Tritiated Water Storage Tank (TWST) system to supplement existing radwaste capacities. The cost of over $20-million was entirely covered by the Department of Energy (DOE), rather than TVA, and they have committed to implementing a similar system at Sequoyah if production is ever expanded to the site. Licensing a commercial reactor for military production has also resulted in costly political liabilities. Due to the new license status for the two reactors at Sequoyah, in 1999 Mitsubishi of Japan refused the sale of a steam generator, which forced the DOE to pay the $200,000 difference required to purchase from a Korean supplier.
Indeed, even if supply is made available from military production pipelines, the price may be a problem. One tritium supply analysis from 2018 compares Canadian commercial prices of ~$25,000/g with tritium produced at Watts Bar at $40,000-$60,000/g. Some important caveats on the latter estimate are that the numbers are based on unclassified approximations in a U.S. Government Accountability Office (GAO) report from 2000 and are not adjusted for inflation. Two facts would lead me to suspect that the higher end of the range is closer to actual costs: (1) tritium production at Watts Bar has scaled much more slowly than initially planned and (2) known incidental costs, as noted above, are not insignificant, and they add up. Still, comparative costs may become increasingly irrelevant as heavy-water produced tritium tapers off because CANDU reactors reach end-of-life. If light water production is the only option, it is the lowest price.
A notable disarmament benefit of tritium production in civilian reactors, already seen at TVA, is the United States' commitment to only use domestic-origin reactor fuel while tritium is being produced. Since the United States has not had domestic enrichment capabilities since 2013, it has chosen to downblend some of its own stockpiles of HEU. As Wang notes, since the end of the Megatons-to-Megawatts program the United States has only converted “a scant seven of its more than 585 metric tons of bomb-grade HEU” from 2013-2016. However, the United States plans to downblend an additional 20 metric tons between 2019-2025 in support of tritium production.
The U.S. Department of Defense tritium production requirements are currently set at 2,800g/18-months. If we estimate that this target represents steady-state requirements based solely on the replacement of inventory lost due to tritium decay, then the total U.S. tritium inventory for nuclear weapon use would be ~35kg. Of the four reactors currently licensed to produce tritium, only the two reactors at Watts Bar are being used to meet this demand. Adding the other two TVA reactors at the Sequoyah site could readily double tritium production. There are dozens of similarly designed reactors that could conceivably produce tritium as well, but they would require additional technical evaluations, public-private acquisition agreements, and updated facility licenses with the Nuclear Regulatory Commission (NRC). Even so, production could not scale by indiscriminately adding more reactors because a bottleneck would still exist once the nation’s sole Tritium Extraction Facility (TEF) at the Savannah River Site (SRS) reached capacity. Although that capacity is unknown, it is not unrealistic for U.S. tritium production in fission reactors to scale up to 5,600g/18-months without significant infrastructural changes.
To be certain, Clery’s and Katwala’s analyses are based on data from 2018. Since then, the ITER timeline has been pushed back. Moreover, other assumptions in the data may also change. However, once ITER and the growing number of fusion startups begin to make significant withdrawals from that reserve, supplies may yet reach the projected ~5kg nadir. In any scenario, doubling U.S. military production capacity could make a significant contribution to these dwindling stockpiles. An additional 2,800g/18-months could extend the peak and help flatten the curve as ITER begins consuming ~1kg/year. With ITER using a significant portion of these reserves, other fusion research projects will be competing for an increasingly scarce resource. However, as long as a fusion reactor can breed almost all of its own tritium, the fuel gap can conceivably be met or significantly mitigated with civilian nuclear fleets.
Second, in addition to bridging the gap to fusion reactor sustainability, there are strategic and national security advantages to providing a military-origin tritium hedge for peaceful fusion supply chains. In a 2011 paper, Rob Goldston makes an effective dual-pronged argument that fusion power will be necessary if the world wants to meet decarbonization while lowering nuclear proliferation risks by reducing reliance on nuclear fission power and associated dual-use technologies. While the U.S. Military continues to stress the national security dimensions of climate change, any technology that can both aid decarbonization and support nuclear nonproliferation would be appealing even if it meant that modest tritium reserves would need to be made available as a hedge for civilian fusion power. However, a transition to fusion also has nonproliferation drawbacks. Goldston and Glaser have addressed some of these risks here and here. In a world with a vastly expanded tritium supply, it will become even more essential to develop robust controls to verify and monitor its production, use, and trade.
Third, nuclear stockpile reductions, while not required for an initial Gigatons-to-Gigawatts strategy, will become complementary to program goals in the future. With Russia’s nuclear saber rattling over its war of aggression in Ukraine, China’s growing nuclear arsenal, the UK’s increasing stockpile threshold for maintaining “minimum deterrence,” not to mention escalating nuclear provocations from Iran and North Korea, additional stockpile reductions in the near-term may be entirely unrealistic. However, NWS should not wait for a geopolitical environment more conducive to disarmament before establishing desirable policies and technical mechanisms for advancing disarmament. Having in place a Gigatons-to-Gigawatts program will provide significant added value to any future stockpile reduction agreements. The benefits would at least be two-fold: (1) countries could assert technological leadership by providing critical fuel materials in support of a nascent fusion industry vanguard and (2) nuclear security realists reluctant to reduce stockpiles might be assuaged by maintaining tritium production capacities that could be redirected if required for national security. While some may bristle at considering the latter as a benefit, it reflects the inconvenient reality of tritium’s inherent dual-use capability.
An additional benefit of utilizing civilian nuclear fleets is that it would also provide a feasible transition away from aging military production reactors in nuclear weapon possessor states. Because these production reactors typically produce weapons-usable plutonium alongside tritium, their decommissioning would also be a meaningful step toward irreversible disarmament. Once nuclear weapon possessing states have sufficient plutonium stockpiles, they maintain such production reactors exclusively to meet ongoing tritium production requirements. This is precisely the case presented by Alex Glaser and Julien de Troullioud de Lanversin in their 2021 paper on the Israeli Dimona reactor.
Just as tritium’s material properties fundamentally differ from those of uranium, so too would an analogous military-to-civilian transfer program. Whereas Megatons-to-Megawatts provided a peaceful path to effectively eliminate a discrete stockpile of HEU, Gigatons-to-Gigawatts would be better conceptualized as a function of production capacity, adding another quantifiable layer to the tritium reserve requirements. The key to the success of any such program would be to maintain sufficient margins to raise or lower tritium production targets as needed. Emergent tritium demand from the implementation of novel fusion technology could additionally be offset by future disarmament treaties, however, such offsets should not be expected or required to meet program goals.
Another important distinction is that Gigatons-to-Gigawatts would not require bilateral or multilateral agreements; instead, it could be successfully enacted unilaterally. A national commitment to a future for fusion fuel would create competitive advantages in technological leadership and energy security. The world is currently witnessing the liabilities inherent in an overreliance on autocratic regimes such as Russia for energy and technology transfer. New national energy paradigms are being constructed in response to Russia’s war in Ukraine, and the nuclear industry must reduce Russia’s prominence and influence. Just as the United States is currently expanding domestic uranium supply and enrichment capabilities, it can also begin to establish the necessary frameworks for tritium independence which will also concomitantly produce a favorable environment for essential research and development work.
Conclusion
In Vannevar Bush’s report to President Roosevelt, “Science, the Endless Frontier,” issued during the final months of World War II, he imagines civilian futures for postwar science amidst great uncertainty and turmoil. Current conditions or assumptions should not dictate or constrain our possible futures. For many, nuclear weapons complexes, perceived as undergirding national and global security, may appear sacrosanct, but when the history of military-to-civilian technology and material transfer is considered, the boundaries are more porous than commonly assumed. If the ultimate goal of nuclear disarmament, enshrined in Article VI of the Treaty on the Nonproliferation of Nuclear Weapons (NPT), is to be achieved, we must begin to act not only as if it is possible but expected. Along with its other benefits, Gigatons-to-Gigawatts could also be seen as a good faith effort to fulfill NPT obligations.
To be sure, fusion may never be commercially viable. Despite recent promising breakthroughs, utility-scale fusion may prove to be excessively complex, prohibitively expensive, or simply too unreliable to leave the lab. However, if these complexities are resolved, sufficient capital is raised, and the necessary technologies are proven reliable, and yet no efforts have been made to address tritium supply concerns that will come sooner or later, a fusion industry would never get off the ground. Let us not allow fusion to fail because we are unable to consider repurposing military tritium for peaceful purposes.
Acknowledgments
I would like to thank two anonymous reviewers for their patience and foresight in offering constructive feedback on an early draft of this paper. Also, much thanks to Rob Goldston who provided invaluable feedback and saved me from making several technical missteps throughout. Alex Stark and Maresa Strano expertly facilitated the review process and provided keen editorial insight into further refining the text. A special thank you to my advisor, Sonja Schmid, who encouraged and now shares my interest in tritium. Thanks to the rest of my NFWG cohort and our discussants Francesca Giovanni, Jenny Town, Kennette Benedict, Sara Kutchesfahani, Jon Wolfsthal, and Peter Scoblic, who challenged and supported our futures. Last but not least, I am indebted to my forever editor-in-residence partner, Rebecca Shelton, who always makes the time to read another draft, and I’m always glad she did.